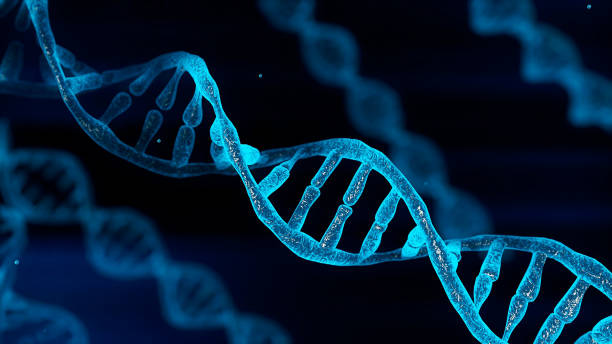
In the grand theatre of biology, DNA plays the starring role, holding the secrets to the script of life itself. Deoxyribonucleic acid, or dna, is the hereditary material in humans and almost all other organisms, acting as the master blueprint from which all biological instructions are derived. This comprehensive exploration delves into the structure, function, and significance of DNA, unraveling its pivotal role in genetics, evolution, and biotechnology.
What is DNA?
DNA, or deoxyribonucleic acid, is the molecular blueprint of life, encoding the genetic instructions vital for the development, function, reproduction, and inheritance of all living organisms and many viruses. This remarkable molecule is composed of two long strands that coil around each other to form a double helix, comprising units called nucleotides. Each nucleotide contains one of four nitrogenous bases—adenine, thymine, cytosine, or guanine—that pair specifically (A with T, and C with G) across the two strands, forming the steps of the helical ladder. These base pairs encode genetic information, which is translated into proteins, the workhorse molecules that perform countless functions within living cells. DNA’s role in heredity, evolution, and many biological processes is central, making it a fundamental substance of life itself.
The Double Helix:
Unveiling DNA’s Structure
The unveiling of DNA’s structure is one of the most groundbreaking achievements in the field of biology and genetics. Before the 1950s, the molecular composition of DNA was a mystery, and its role in heredity was only beginning to be understood. The discovery of DNA’s structure marked a pivotal moment in science, shedding light on the molecular basis of life.
DNA’s structure is famously characterized by the double helix model, first proposed by James Watson and Francis Crick in 1953. This model was based on the X-ray diffraction images produced by Rosalind Franklin and the information that the molecule contains a repeating sugar-phosphate backbone with nitrogenous bases projecting inward. The double helix resembles a twisted ladder, where the sugar and phosphate molecules form the sides, and pairs of nitrogenous bases (adenine paired with thymine and cytosine paired with guanine) form the rungs.
The significance of the base pairing is profound: it explains how DNA replicates and how genetic information is encoded and passed on to subsequent generations. The sequence of the bases along the DNA strand constitutes the genetic instructions for the synthesis of proteins, which are crucial for the structure and function of living organisms.
Moreover, the discovery of the double helix opened the floodgates for molecular biology. It led to an understanding of how genes are copied and expressed, how mutations arise, and how the genetic code is translated into proteins. It laid the foundation for modern biotechnological advances, including DNA sequencing, genetic engineering, and forensic science.
The unveiling of DNA’s structure was not just a milestone in understanding the chemical basis of heredity; it was a revolution that transformed biology into a molecular science, offering insights into the very essence of life itself.
DNA Replication: The Art of Precision
DNA replication is a meticulously precise process that allows cells to duplicate their genetic material before dividing. This intricate mechanism ensures that each new cell inherits an exact copy of the DNA, which is vital for the maintenance of genetic information across generations.
The process of dna replication begins at specific sites on the DNA molecule known as origins of replication, where the double helix unwinds to expose the two strands. This unwinding is facilitated by enzymes called helicases, which break the hydrogen bonds between the base pairs, separating the two strands and forming a “replication fork.”
Building on the exposed strands, a group of enzymes called DNA polymerases add new nucleotides to each original strand. These nucleotides are matched with their complementary bases on the original strands (adenine with thymine, and cytosine with guanine), creating two new strands that are identical to the original ones. Because each new DNA molecule consists of one old strand and one new strand, this process is known as semi-conservative replication.
In summary, DNA replication is a fundamental biological process, characterized by its precision and efficiency, ensuring the continuity of life by accurately transmitting genetic information from one cell generation to the next.
From DNA to Proteins: The Central Dogma
The Central Dogma of molecular biology is a framework that explains the flow of genetic information within a biological system. Articulated by Francis Crick in 1958, it delineates the process by which the information in DNA is translated into proteins, the functional molecules within the cell. This dogma outlines two main steps: transcription and translation.
dna structure
Transcription: From DNA to RNA
Transcription is the first step, where the DNA sequence of a gene is transcribed to produce a complementary RNA molecule. This process is facilitated by the enzyme RNA polymerase, which unwinds the DNA and synthesizes a single-stranded RNA copy. The RNA transcript is a messenger RNA (mRNA) molecule that carries the genetic information from the DNA in the nucleus to the ribosomes, the cell’s protein factories, located in the cytoplasm.
RNA Processing
In eukaryotic cells, the primary mRNA transcript undergoes processing before it exits the nucleus. This includes the addition of a 5′ cap and a poly-A tail, as well as the removal of non-coding regions called introns through a process known as splicing. The resulting mature mRNA strand contains only the coding regions or exons, ready to be translated into a protein.
Translation: From RNA to Protein
Translation is the process by which the mRNA is decoded by a ribosome to build a protein. The mRNA sequence is read in sets of three nucleotides, known as codons, each specifying a particular amino acid (the building blocks of proteins). Transfer RNA (tRNA) molecules bring the appropriate amino acids to the ribosome based on the codon sequence. The ribosome facilitates the bonding of these amino acids, creating a growing polypeptide chain that eventually folds into a functional protein.
Significance of the Central Dogma
The Central Dogma is fundamental to understanding biological processes. It explains how the genetic information stored in DNA dictates the structure and function of proteins, which carry out most of the work in cells. This flow of information—DNA to RNA to protein—is crucial for the development, functioning, and reproduction of all living organisms.
Understanding the Central Dogma has profound implications for various fields, including genetics, biochemistry, and medicine, providing insights into how genetic information is expressed and how it can be influenced or altered in cases such as genetic disorders or cancer.
Genetic Variation and Inheritance
Genetic variation and inheritance are foundational concepts in the field of genetics, providing insights into how traits are passed from parents to offspring and how species evolve over time. These variations are crucial for the survival and adaptation of species, enabling populations to respond to changes in the environment.
Genetic Variation
Genetic variation refers to the diversity in gene frequencies within a population. This variation arises through mutations, which are changes in the DNA sequence, recombination during sexual reproduction, and gene flow between populations. Mutations can introduce new gene variants, or alleles, into a population. Recombination, which occurs during meiosis, shuffles alleles and produces new combinations of genes, contributing to genetic diversity. Gene flow, the transfer of alleles between populations, can also introduce new genetic variations.
Inheritance
Inheritance is the process by which genetic information is passed on from one generation to the next. This is fundamentally governed by the rules of Mendelian genetics, established by Gregor Mendel in the 19th century. Mendel’s laws— the law of segregation and the law of independent assortment—describe how alleles are distributed in gametes and how they combine during fertilization.
- Law of Segregation: This law states that during gamete formation, the two alleles for each gene segregate from each other, so that each gamete carries only one allele for each gene.
- Law of Independent Assortment: This law explains how alleles of different genes are distributed independently of one another from parent to offspring.
Some traits are influenced by multiple genes (polygenic inheritance) or involve interactions between genes and the environment. Additionally, some traits do not follow Mendelian patterns of inheritance, such as those linked to chromosomes (sex-linked traits) or influenced by mitochondrial DNA.
DNA and Evolution
DNA plays a pivotal role in the process of evolution. Serving as the molecular foundation for genetic variation and the transmission of traits across generations. Evolution is the change in the heritable characteristics of biological populations over successive generations. Is fundamentally driven by the mechanisms of mutation, natural selection, genetic drift, and gene flow, all of which involve changes at the dna test level.
Mutation: The Source of Genetic Variation
Mutations are changes in the DNA sequence that can occur spontaneously during DNA replication or be induced by environmental factors. While many mutations are neutral or deleterious, some can confer advantages to organisms in specific environments. Allowing these genetic changes to be passed on to future generations. Over time, this can lead to the emergence of new traits and even new species. Illustrating the central role of DNA in the diversification of life on Earth.
Natural Selection: The Driving Force of Evolution
It acts on the genetic variation within a population, with DNA serving as the substrate for this variation. Organisms with advantageous genetic traits are more likely to survive and reproduce, thereby passing these beneficial traits to their offspring.
Genetic Drift: Random Changes in Gene Frequency
In a population due to random sampling of organisms. This mechanism can cause significant changes in a population’s genetic structure, particularly in small populations. Where chance events can lead to the loss or fixation of alleles, independent of their influence on survival and reproduction.
Gene Flow: The Exchange of Genes Between Populations
Gene flow, or genetic migration, occurs when organisms. Or their gametes move from one population to another, introducing new genetic material. This process can increase genetic variation within a population and reduce differences between populations. Potentially countering the effects of natural selection and genetic drift.
DNA’s Role in Tracking Evolutionary History
Beyond its role in the mechanisms of evolution. DNA is also a crucial tool for understanding the evolutionary history of life. Comparative genomics, the study of the similarities and differences in DNA sequences between different organisms. Provides insights into their evolutionary relationships, revealing how species have diverged and evolved over millions of years. Molecular clocks, which estimate the time of evolutionary events based on the rate of genetic mutations. Further illustrate how DNA serves as a historical record of evolution.
Biotechnology and Genetic Engineering
Biotechnology and genetic engineering are fields that leverage our understanding of DNA. And biological processes to develop technologies and products that improve lives, industries, and the environment. Through the manipulation and application of living organisms or their components. Biotechnology has profound impacts across medicine, agriculture, and industrial processes.
Genetic Engineering:
Genetic engineering, a subset of biotechnology, involves directly manipulating the DNA of organisms to alter their characteristics or capabilities. This manipulation often includes adding, deleting. O modifying genes within an organism’s genome to achieve desired traits or produce specific biological products.
Applications in Medicine:
In medicine, genetic engineering has led to revolutionary treatments and diagnostic tools. It enables the production of recombinant proteins, such as insulin for diabetes treatment, previously harvested from animal sources. Gene therapy, another application, involves altering the genes within an individual’s cells to treat or prevent disease. This approach has shown promise in treating genetic disorders, certain types of cancer, and viral infections.
Agricultural Innovations:
In agriculture, genetic engineering has produced genetically modified organisms (GMOs). That exhibit traits such as increased yield, pest resistance, and drought tolerance. Crops like Bt corn, engineered to express a bacterial toxin harmful to specific pests, and Roundup Ready soybeans. Designed to resist herbicides, are examples of how genetic engineering can enhance food security and farming efficiency.
Industrial Biotechnology:
Industrial biotechnology uses enzymatic processes and microorganisms to produce biofuels, reduce waste, and create more sustainable manufacturing processes. For instance, genetically engineered bacteria are used to clean up oil spills through bioremediation. Demonstrating how biotechnology can address environmental challenges.
Ethical and Societal Considerations:
While biotechnology and genetic engineering offer significant benefits, they also raise ethical and societal concerns. Issues such as GMO food safety, the potential for gene-edited organisms to impact ecosystems. And the ethics of human genetic modifications are subjects of ongoing debate. It is crucial to balance the potential benefits with considerations of ecological impact, bioethics, and regulatory oversight.
Conclusion: The Ongoing Saga of DNA
The exploration of DNA is far from over. Each discovery peels back a layer, revealing more questions and deepening our awe of the complexity of life. As we continue to decipher the language of DNA, we edge closer to understanding the very essence of biological existence. Harnessing its power to innovate and exploring its potential to heal. DNA, the blueprint of life, remains one of nature’s most profound mysteries, a molecule that encapsulates the past. Defines the present, and shapes the future of all living beings.