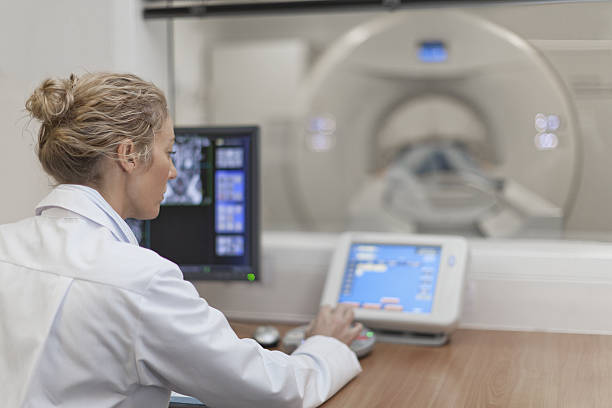
Magnetic Resonance Imaging (MRI) is a revolutionary medical imaging technology that has transformed the field of diagnostic medicine. Since its inception in the early 1970s, MRI has become an indispensable tool for physicians and researchers, providing unparalleled insights into the human body’s inner workings. In this comprehensive article, we will delve into the world of MRI, exploring its history, principles, applications, and future prospects of this remarkable technology.
I. A Brief History of MRI
MRI’s roots can be traced back to the mid-20th century when physicists and scientists were exploring the principles of nuclear magnetic resonance (NMR). NMR, a fundamental concept in atomic and molecular physics, was initially developed to study the magnetic properties of atomic nuclei. However, it was not until the 1970s that MRI, a medical imaging application of NMR, was developed. The pioneers in this field include:
- Paul Lauterbur (1973): Paul Lauterbur, an American chemist and biophysicist, is often credited with the invention of MRI. He introduced the idea of spatially encoding NMR signals, which led to the creation of the first two-dimensional MRI image. Lauterbur’s work laid the foundation for the development of modern MRI techniques.
- Sir Peter Mansfield (1977): Sir Peter Mansfield, a British physicist, further advanced MRI technology by developing techniques for rapid imaging and three-dimensional reconstruction. His contributions were critical in making MRI practical for clinical use.
These early breakthroughs in MRI technology paved the way for its widespread adoption in medicine. In 2003, Paul Lauterbur and Sir Peter Mansfield were jointly awarded the Nobel Prize in Physiology or Medicine for their pioneering work in the development of MRI.
II. The Principles of MRI
At its core, MRI relies on the principles of nuclear magnetic resonance (NMR) to produce detailed images of the human body’s internal structures. NMR is based on the behavior of atomic nuclei in the presence of a strong magnetic field and radiofrequency (RF) pulses. Here’s how MRI works:
- Magnetic Fields: MRI machines utilize powerful magnets to generate a strong, static magnetic field within the imaging area. The main magnetic field aligns the hydrogen nuclei (protons) in the body along its direction.
- RF Pulse: When a brief RF pulse is applied at the resonant frequency of the protons (typically the hydrogen nuclei), it temporarily flips the protons’ magnetic moments away from the main magnetic field.
- Relaxation: After the RF pulse is turned off, the protons gradually return to their aligned state parallel to the main magnetic field. This process involves two types of relaxation: T1 (spin-lattice relaxation) and T2 (spin-spin relaxation). These relaxation times are characteristic of different tissues and play a crucial role in image contrast.
- Signal Detection: As the protons relax, they emit radiofrequency signals in response to their realignment with the main magnetic field. These signals, known as free induction decay (FID), are detected by RF coils placed around the patient.
- Image Reconstruction: The detected signals are processed by a computer to create detailed cross-sectional images (slices) of the body. Different tissues produce varying signal intensities, resulting in image contrast.
- Spatial Encoding: To obtain 3D information, MRI uses gradient magnetic fields to vary the magnetic field’s strength across the imaging area. By changing these gradients, MRI can differentiate spatial locations and produce detailed, high-resolution images.
III. MRI Techniques and Modalities
MRI offers a wide range of imaging techniques and modalities that can be tailored to specific clinical needs. Some of the most common MRI techniques and modalities include:
- T1-Weighted Imaging: T1-weighted images are useful for distinguishing between different types of tissues in the body. This technique provides excellent anatomical detail and is often used for brain and musculoskeletal imaging.
- T2-Weighted Imaging: T2-weighted images highlight differences in tissue water content and are particularly useful for detecting pathological conditions, such as tumors and inflammation.
- Functional MRI (fMRI): Functional MRI is a specialized technique that measures changes in blood oxygenation levels to map brain activity. It is widely used in neuroscience and cognitive research.
- Diffusion-weighted imaging (DWI): DWI assesses the random motion of water molecules in tissues. It is invaluable for detecting and characterizing diseases like stroke and tumors.
- Magnetic Resonance Angiography (MRA): MRA is used to visualize blood vessels in the body, helping diagnose conditions like aneurysms and arterial blockages.
- Magnetic Resonance Spectroscopy (MRS): MRS measures the chemical composition of tissues by analyzing the resonance frequencies of specific metabolites. It is commonly used to study brain disorders.
- Magnetic Resonance Elastography (MRE): MRE is employed to assess tissue stiffness and detect liver fibrosis, among other applications.
- Cardiac MRI: Cardiac MRI provides detailed images of the heart and is crucial for evaluating heart structure and function. It is used in diagnosing heart diseases and planning surgical interventions.
IV. Clinical Applications of MRI
MRI’s versatility and non-invasive nature make it an essential tool in a wide range of clinical applications. Some of the most common medical uses of MRI include:
- Neuroimaging: MRI is extensively employed to study the brain and spinal cord, aiding in the diagnosis of neurological conditions such as multiple sclerosis, brain tumors, and stroke.
- Orthopedics: Musculoskeletal MRI is vital for assessing joint injuries, ligament tears, and bone fractures. It is instrumental in planning orthopedic surgeries and monitoring treatment outcomes.
- Abdominal and Pelvic Imaging: MRI can visualize the liver, kidneys, pancreas, and other abdominal organs, helping diagnose conditions like liver tumors, kidney disease, and pelvic disorders.
- Breast Imaging: Breast MRI is a powerful tool for breast cancer screening and detection, especially in high-risk patients.
- Oncology: MRI is an essential component of cancer diagnosis and treatment planning. It helps locate tumors, assess their size and extent, and monitor the effectiveness of cancer treatments.
- Cardiovascular Imaging: Cardiac MRI aids in the evaluation of heart diseases, including congenital heart defects, coronary artery disease, and myocardial infarctions.
- Obstetrics: MRI can be used during pregnancy to assess fetal development and detect anomalies, although it is not as commonly used as ultrasound for this purpose.
- Angiography: MRA is employed for imaging blood vessels, aiding in the diagnosis and treatment of vascular conditions.
V. Advantages of MRI
MRI offers numerous advantages that have cemented its position as a preferred imaging modality in many clinical scenarios:
- Non-Invasiveness: MRI is a non-invasive imaging technique, which means it does not involve ionizing radiation like X-rays or CT scans. This makes it safer for patients, especially when repeated imaging is required.
- Superior Soft Tissue Contrast: MRI excels at visualizing soft tissues, making it ideal for detecting tumors, assessing organ function, and differentiating between normal and abnormal tissue.
- Multi-Planar Imaging: MRI can produce images in multiple planes (axial, sagittal, coronal), providing a comprehensive view of the anatomy and facilitating accurate diagnosis.
- Functional Imaging: With techniques like fMRI and MRS, MRI can provide insights into tissue function and chemical composition, not just anatomy.
- Versatility: MRI can be customized with various sequences and contrast options to suit different clinical needs, from brain imaging to cardiac assessments.
- Safety: The absence of ionizing radiation means MRI can be used for pediatric and pregnant patients without concern for radiation exposure.
- Real-Time Imaging: Advances in MRI technology have allowed for real-time imaging, which is valuable in procedures like MR-guided interventions and surgery.
VI. Limitations and Challenges
While MRI is a powerful imaging tool, it also has some limitations and challenges that need to be considered:
- High Cost: MRI equipment is expensive to purchase, install, and maintain, which can make it less accessible in certain healthcare settings.
- Long Scan Times: Some MRI exams can take a considerable amount of time, which may be challenging for patients with claustrophobia or conditions that make lying still difficult.
- Metal Interference: The strong magnetic field of an MRI machine can be dangerous for patients with certain metallic implants or foreign bodies. It can also affect the quality of images in the presence of metal.
- Noise: MRI scans are loud due to the rapid switching of gradient coils. Patients are typically provided with ear protection to minimize discomfort.
- Limited Availability: In some regions, access to MRI facilities may be limited, leading to long waiting times for non-urgent cases.
- Contraindications: Some patients with certain medical conditions, such as severe kidney disease, may be contraindicated for contrast-enhanced MRI.
- Image Artifacts: Various factors, including patient motion and magnetic field inhomogeneities. Can lead to image artifacts that may affect diagnostic accuracy.
VII. The Future of MRI
The future of MRI holds exciting possibilities, driven by ongoing research and technological advancements. Some key developments to watch for include:
- Higher Field Strengths: MRI machines with stronger magnetic fields (7 Tesla and beyond) are being developed, offering improved image quality and more detailed information.
- Artificial Intelligence (AI): AI and machine learning are being integrated into MRI data analysis, aiding in automated disease detection and image enhancement.
- Functional Imaging Advances: Functional MRI techniques continue to evolve, providing deeper insights into brain activity and cognitive function.
- Personalized Medicine: MRI will play a crucial role in the emerging field of personalized medicine. Tailoring diagnostic and treatment approaches to an individual’s unique physiology.
- Hybrid Imaging: Combining MRI with other imaging modalities like positron emission tomography (PET) is becoming more common. Allowing for comprehensive anatomical and functional assessments.
- Point-of-Care MRI: Portable and point-of-care MRI systems are being developed, which could bring MRI technology to remote or underserved areas.
- Improved Patient Experience: Efforts to reduce scan times, and make MRI machines less noisy. Enhancing patient comfort will continue to improve the overall patient experience.
Conclusion
Magnetic Resonance Imaging (MRI) has revolutionized diagnostic medicine, offering unparalleled insights into the human body’s anatomy and function. With its non-invasive nature, excellent soft tissue contrast, and versatility. MRI has become an indispensable tool for healthcare professionals in a wide range of specialties. As technology continues to advance. The future of MRI holds the promise of even more accurate and personalized diagnostic and therapeutic capabilities. Further enhancing its significance in the field of medicine.